Introduction
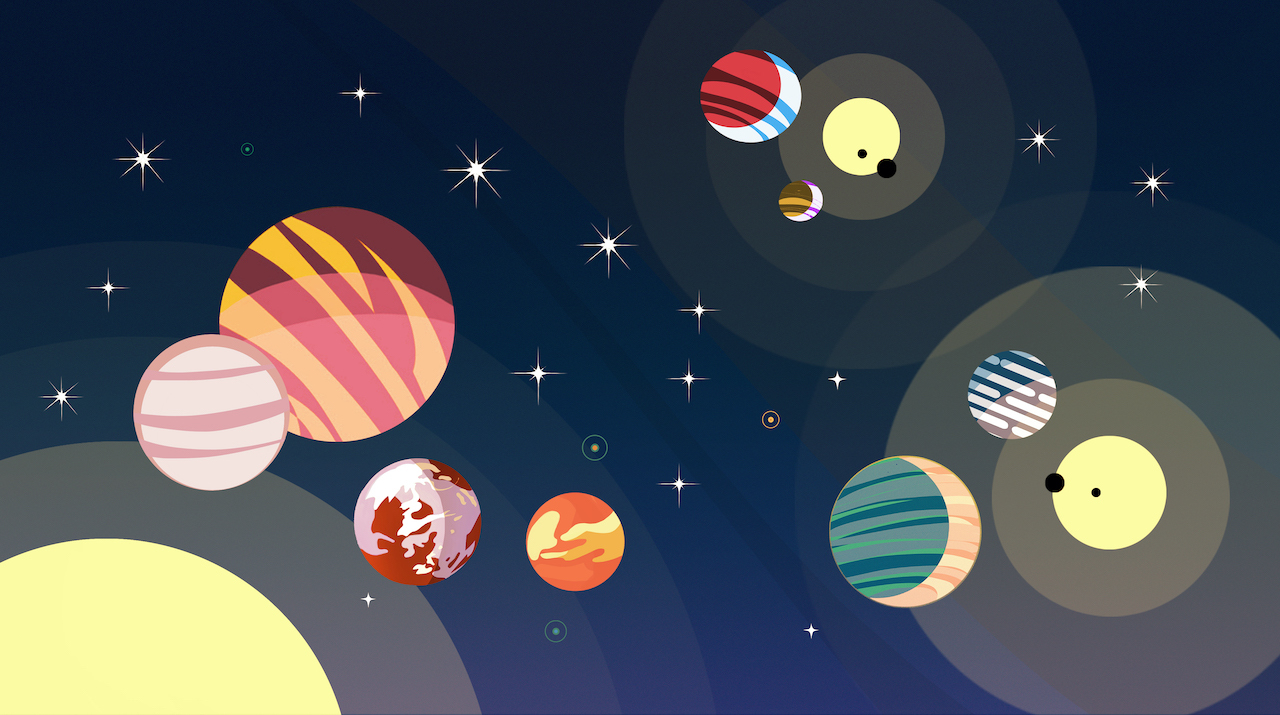
Image Source: NASA/JPL-Caltech/Lizbeth B. De La Torre
Planets outside our solar system are called exoplanets. The first exoplanets were discovered in the 1990’s, and since then we’ve confirmed more than 4300 as of this writing. While we’ve only begun to scratch the surface of all the exoplanets in the galaxy, we’ve already uncovered a beautiful diversity among alien worlds. The first exoplanets discovered were hot Jupiters, gaseous planets in extremely close orbits around their stars. One year for hot Jupiters may just be a few days, and the hottest can be upwards of 4000 Kelvin (the surface of the sun is 5600 Kelvin). Next are sub Neptunes, which as their name suggests, are also gaseous planets but which are smaller than Neptune. These two types of planets are peculiar as there are none like them in our own solar system.
Of course, there are also terrestrial planets, rocky like the Earth. These can also be quite extreme, with liquid magma oceans or stationary hurricanes on permanent daysides. However, we know of some that orbit within their star’s habitable zone, traditionally defined as where liquid water on the surface could exist. It’s hard to pin down whether a planet is truly habitable as there are many factors at play, like stellar activity or the properties of the planet’s atmosphere.
Atmospheres are very important to the study of alien worlds. They are the most accessible part of an exoplanet we can measure, and they give us clues about what the planet is made of and the conditions on the surface. Exoplanet atmospheres are usually studied with spectroscopy, or counting photons by their different colors. We know certain molecules absorb or emit light at specific colors, so by counting photons at these colors, we can identify which molecules are in an atmosphere. By studying atmospheres, we can learn more about their underlying physics and how planets, including our own, came to be.
High Resolution Cross Correlation Spectroscopy
The Earth’s atmosphere absorbs light strongly in the infrared. This is good for us, as if our atmosphere didn’t absorb light and warm up, the surface of the Earth would actually be below freezing! However, this isn’t quite so good for astronomy because if you point an infrared telescope up at the sky, the spectrum will be dominated by features of our own atmosphere. This might not be the worst thing if you’re studying a bright star, but for exoplanets, which can be millions, if not billions, of times fainter than their star, every photon counts! This is why so much groundbreaking exoplanet science has been done with space telescopes. No atmosphere, no problem!
Space telescopes are not without their drawbacks though. They’re limited by their size, and spectrographs on these telescopes are often low resolution. That is, they can only split light into so many colors, or wavelengths. This can severely limit our ability to study an exoplanet. In contrast, large ground based telescopes are often high resolution, but of course, there’s that atmospheric absorption problem. Well, astronomers have recently found a way around this through a method called high resolution cross correlation spectroscopy.
As it turns out, many of the planets we study are orbiting their stars so fast that we can actually observe the Doppler shift of their spectrum in the course of a few hours. As the planet’s velocity along our line of sight changes, so does the wavelength of its spectral features. This change is very small, but resolvable at high enough spectral resolution. Using the fact that the planet’s spectrum is much fainter than that of the star and is not constant in time, we can separate it from the things we’re not interested in, like the stellar spectrum and the interference from Earth’s atmosphere.
To detect the planet signal and infer properties about its atmosphere, we make models of what we think the atmosphere might look like, then try to match, or cross correlate, it with the data. This is a lot like trying to match up fingerprints in a database. The best match model will have a high correlation value and is very likely to be very close to the actual thing. Several molecules that would otherwise be impossible to find with space telescopes have been detected in exoplanets this way.
Can we see atmospheres on terrestrial planets around small stars?
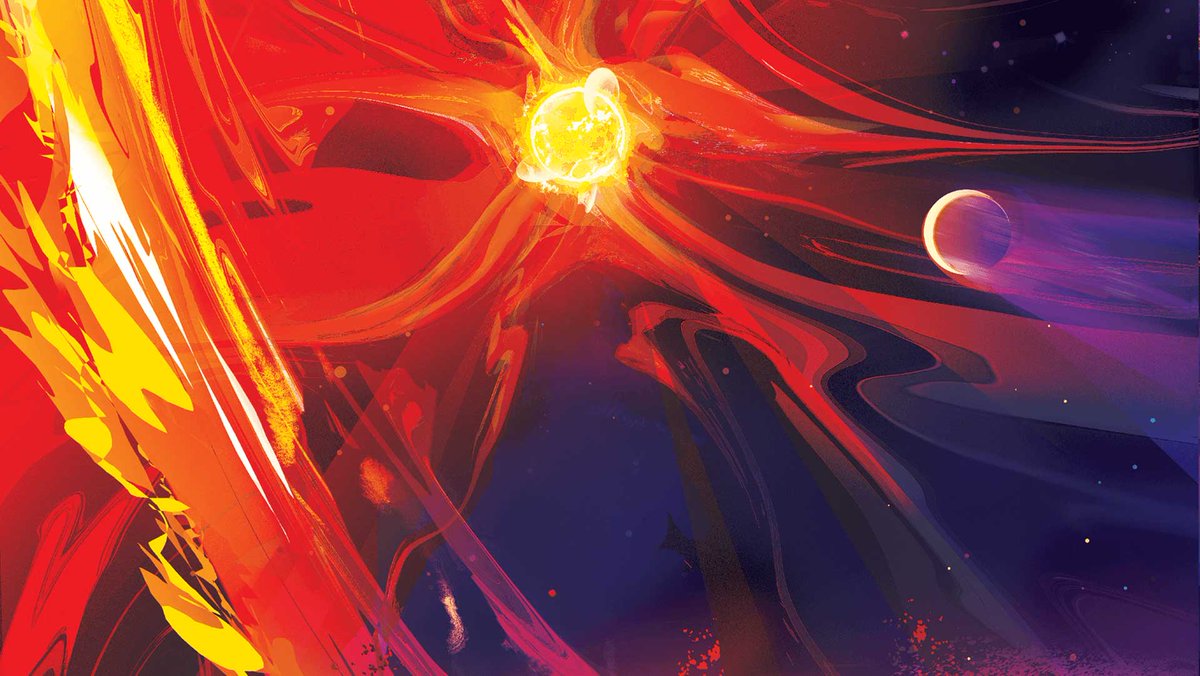
Image Source: NASA-JPL/Caltech
The most common type of star are M-dwarfs. These stars are much smaller and cooler than the sun, but they make up for 70% of all stars in the Milky Way. Because they are the smallest and faintest type of star, M-dwarfs are also the easiest stars to find exoplanets around, especially rocky planets. Does that mean it’s likely we’ll find the first truly habitable alien world orbiting a M-dwarf? Well, it’s complicated.
Because M-dwarfs are so cool, their habitable zone is much closer than compared to other stars. When you get very close to your star though is when you start running into problems. First, a planet in the habitable zone of an M-dwarf is likely to be tidally locked. That means one side of the planet always faces the star, while the other always faces away. Permanent day on one side, permanent night on the other, and eternal twilight between them. Our moon is like this - the same side always faces the Earth. Tidal locking can lead to extreme temperature differences on the planet, and the planet's oceans may evaporate on the dayside while its atmosphere freezes out on the night side.
Additionally, you have to deal with stellar activity. M-dwarfs are like interstellar babies throwing temper tantrums. In their early lives, they have frequent flaring events and high stellar wind. Both of these could blow away the planet’s atmosphere and dessicate the surface. M-dwarfs also have high levels of UV radiation. Be sure to pack some sunscreen!
So, while an exoplanet may be in the M-dwarf habitable zone, its atmosphere may have been destroyed long ago, and most living things need to breathe! However, some of these things might be good for habitability too: UV radiation is crucial to the formation of ozone and other organic compounds, or tidal locking may heat an otherwise too cold planet from the inside. Current observations of rocky planets are lacking, but many exoplanet scientists want to get to the bottom of this. Several rocky planets around cool stars will be observed with the upcoming James Webb Space Telescope. While most of the planets it observes will be much too hot for life (as we know it!), we’ll be able to check whether or not atmospheres can stick around on these kinds of planets. If we find a very hot exoplanet orbiting a M-dwarf that kept its atmosphere after all this time, that bodes well for atmospheres, and by extension life, on cooler planets.